Group Delay Dispersion and White Light Interferometry
Authors: Chase Toncheff, Olivia Wheeler
Group delay dispersion (GDD) refers to a quantity that describes the change of the temporal profile of an ultrafast laser pulse within various optical media. Understanding GDD is critical for understanding how ultrafast laser pulses are stretched or compressed by optical components. GDD has significant implications for applications such as telecommunication and laser systems, where precise pulse shaping is crucial. White light interferometry (WLI) utilizes the interference of light waves to extract information about the applied phase to find the GDD of different optical elements.
Group Delay Dispersion
Group delay dispersion is an optical characteristic that arises in the study of waves and their propagation, particularly in the field of ultrafast laser optics. To understand GDD, let's start with the basics. In physics, waves can be described by their amplitude and frequency. In the case of light waves, different wavelengths, or colors, have different frequencies. However, waves can also have different phases, which determine the position of a particular point during the wave cycle over time. These are both important to understand when measuring or manipulating GDD.
Now, imagine a wave packet, or a collection of different frequencies in one pulse. This wave packet could represent a singular pulse of an ultrafast laser. When this wave packet travels through a medium, such as a fiber optic cable or a prism, it interacts with the material and undergoes a phenomenon called dispersion. This occurs because different frequencies of the wave packet travel at slightly different velocities through the medium. The speed at which light travels is dependent on the material’s index of refraction and index of refraction is dependent on wavelength. As the wave packet moves through a medium, the individual frequencies separate from each other. This is known as chromatic dispersion, and it leads to an elongation of the original pulse shape.
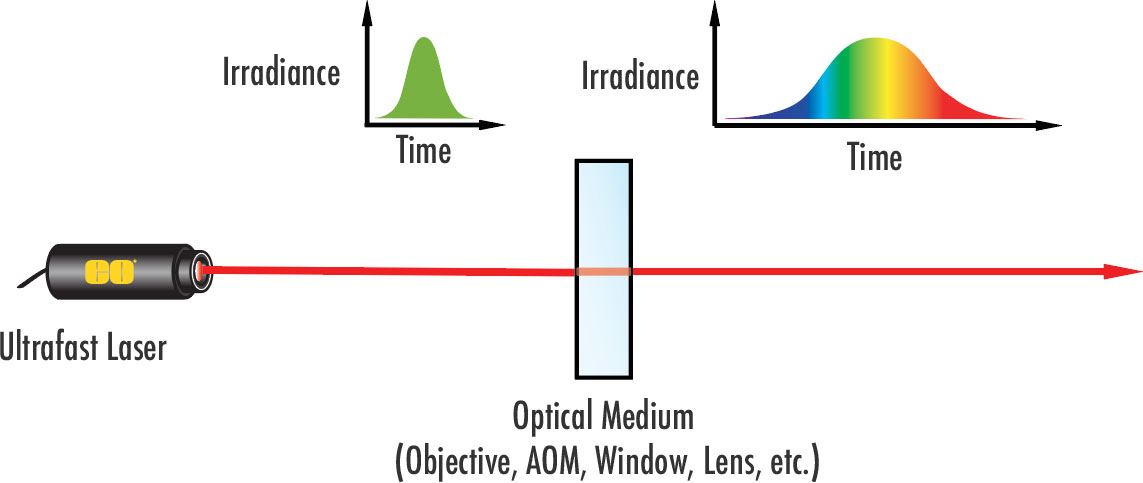
Figure 1: Dispersion leads to the broadening of ultrafast laser pulses.
Group delay dispersion is the characterization of the aforementioned breakdown of original pulse shape, and it tells us how the delay experienced by different frequencies changes within the wave packet due to dispersion. It is the second derivative of a material’s dispersion equation with respect to wavelength, meaning that the units for GDD are commonly fs2 or ps2.1 Moreover, GDD varies across material types, meaning that ultrafast systems are extremely sensitive to material changes; any inconsistencies within a system can led to stretching or compression of the pulse.
White Light Interferometry
The general principle of interferometry is based on the fact that light waves can interfere with each other, leading to constructive or destructive interference depending on the path length difference between the waves.2 In white light interferometry, a broadband light source, such as a white light LED, is used to emit light of various wavelengths simultaneously. The WLI used at Edmund Optics® is based on a Michelson interferometer setup as shown in Figure 2 below. The white light is split into two paths: a reference path and a measurement path. In the reference path, the light is directed towards a reference mirror, which reflects it back towards the detector. In the measurement path, the light is directed towards the optic under investigation. After the test optic, the light is reflected back to the beamsplitter. Some of the light reflects off the beamsplitter, while the rest passes through and interacts with the reference light. These two sets of light waves are recombined and interfere with each other.3
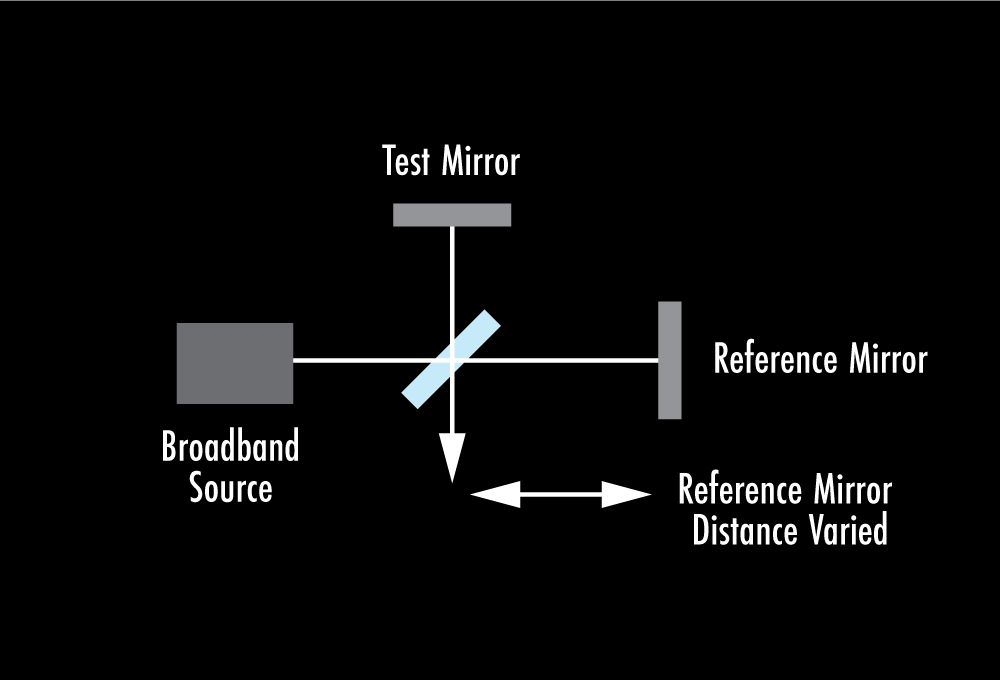
Figure 2: Schematic of a typical white light Michelson interferometer used to determine Group Delay Dispersion. The instrument is kept stationary as the length of the reference arm is varied.
The WLI used by Edmund Optics® is the Gobi ® by UltraFast Innovations. This WLI is a Michelson Interferometer, however the setup used in our labs uses two beamsplitters. The first beamsplitter is the one seen in the standard Michelson Interferometer setup but the other is the exact same beamsplitter further down the system in the reference path. In this case, it is known as a compensating surface, and it is at the exact same angle of incidence as the first beamsplitter. This is because the beamsplitter will induce some amount of dispersion which differs based on whether the light is reflected or transmitted through the beamsplitter. So, by introducing a compensating surface in the test arm, we are able to match the amount of dispersion on both the reference and test arms, allowing for the possibility of interference.
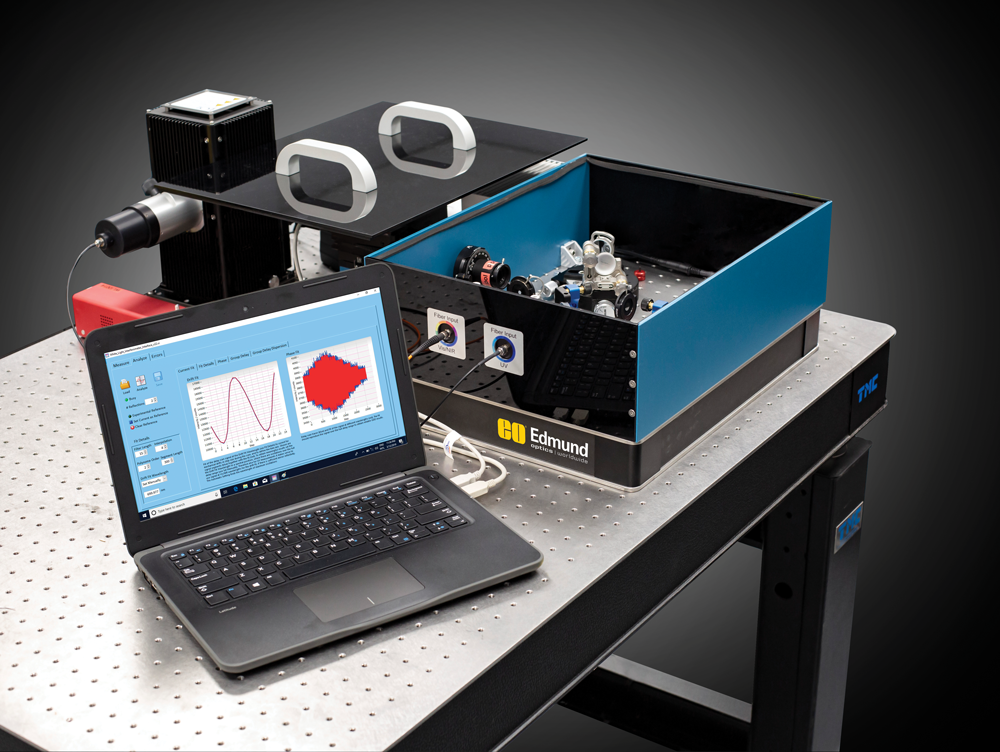
Figure 3: Gobi® White Light Interferometer by UltraFast Innovations
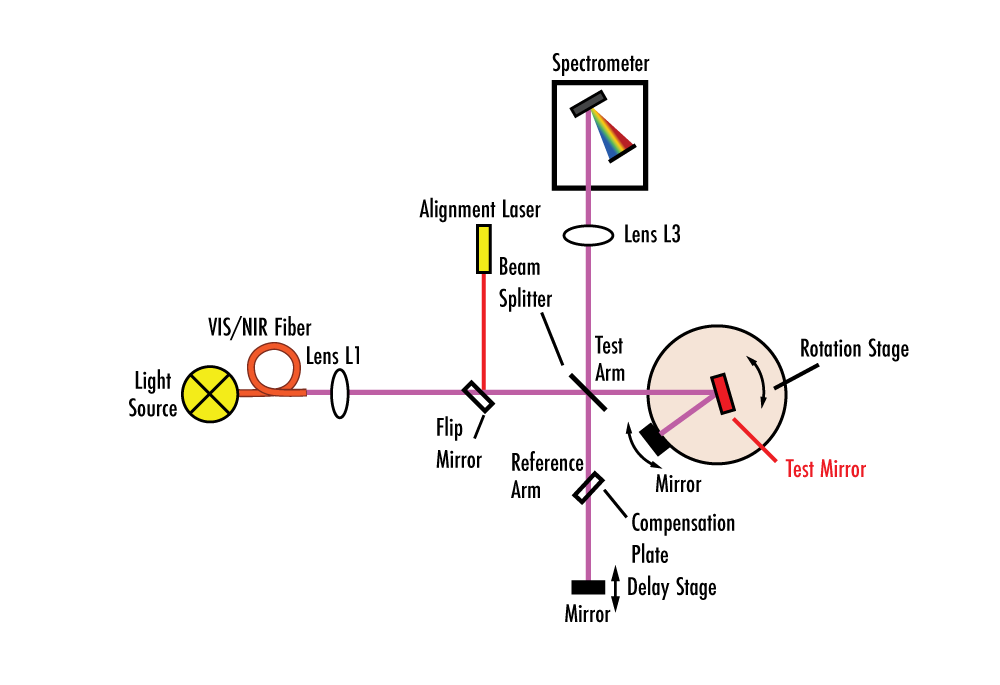
Figure 4: Simplified system drawing of the UltraFast Innovations WLI. This drawing excludes its UV capabilities for simplicity.
The interference patterns generated by the recombined light contain information about the phase difference between the two arms of the interferometer. Since the light source emits a range of wavelengths, each wavelength interferes at a different angle, resulting in a series of interference fringes dependent on wavelength. These fringes are captured by a detector, such as a CCD camera.
One of the most important properties of the WLI to understand is that its measurements contain phase information, not GDD. Determining GDD is done by varying the “time” at which the light in the reference arm is during its phase. This process is called phase-shifting interferometry. The WLI uses a piezoelectric motor to attain nanometer-scale steps for the reference mirror, allowing for high-precision phase measurements. With the acquired interference patterns and known phase shifts, advanced algorithms are then applied to process the phase data and obtain the GDD values across the wavelength range of interest.
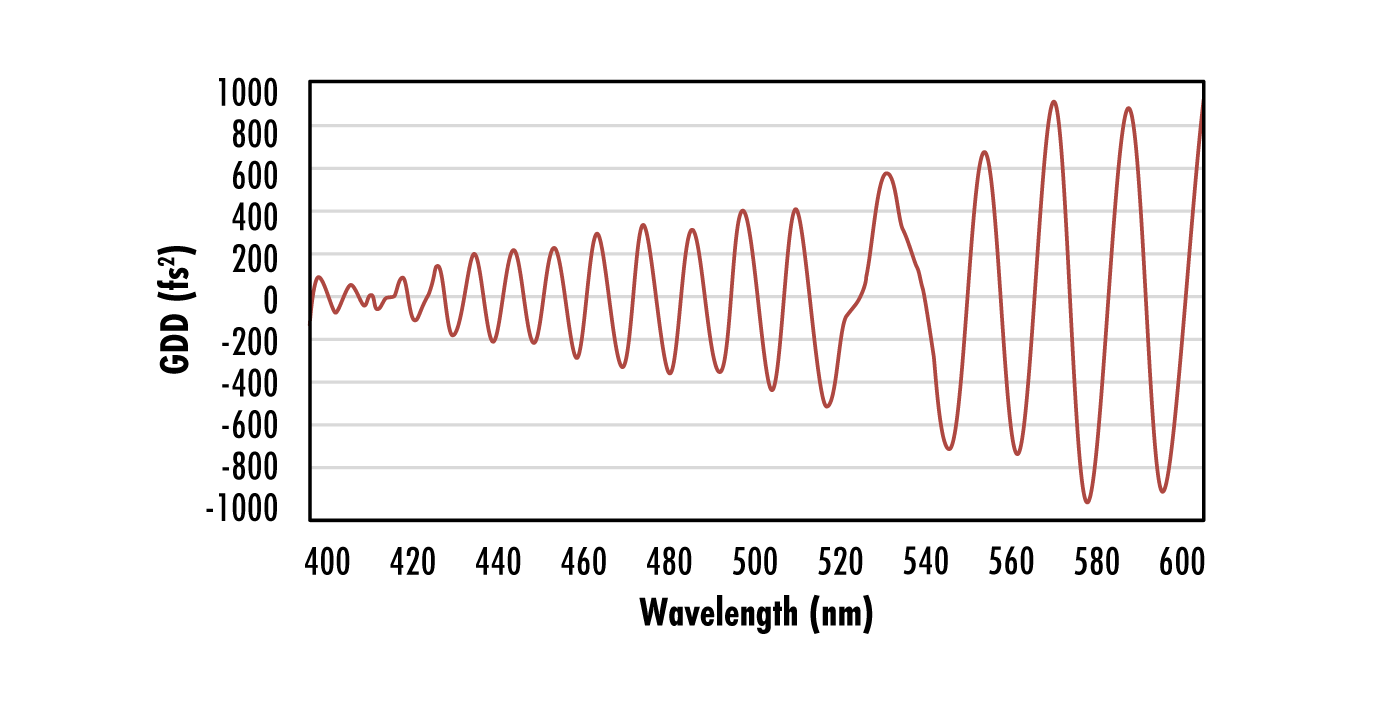
Figure 5: Example GDD results from WLI.
Applications Within Edmund Optics®
Measuring GDD using WLI is useful in various optical applications, especially in the field of ultrafast optics. It allows for the characterization of materials, components, and systems that exhibit dispersion effects, which can significantly degrade the performance of ultrafast laser pulses. By understanding and quantifying GDD, researchers and engineers can optimize optical designs, compensate for dispersion effects, and ensure precise control over the pulse duration and spectral properties of ultrafast lasers.
One of the primary purposes of characterizing the GDD of an optical surface is for coating development. This is how WLI is being used in the Edmund Optics® Laser Optics Center located outside of Tampa, Florida, providing the ability to test different coatings to help simulate systems before they are integrated into ultrafast laser systems. Although GDD is valuable in terms of simulation, oftentimes it is used for quality assurance. GDD can be estimated based on multiple factors including index of refraction and wavelength, but by using WLI it is possible to test and provide evidence that these estimations are true. In both cases, Edmund Optics® is using white light interferometry to expand its understanding and use of dispersion characteristics.
The newest introduction to this principle can be seen in our Ultrafast Pulse Compressors. The goal of these products is to compress the pulse duration using a single-knob system that can be easily introduced into an ultrafast system. They correct for the positive group delay dispersion experienced in most optical media easily by having two planar mirrors compress the pulse back to its original shape. Each reflection imparts some negative GDD that balances out the positive GDD from other parts of the system.
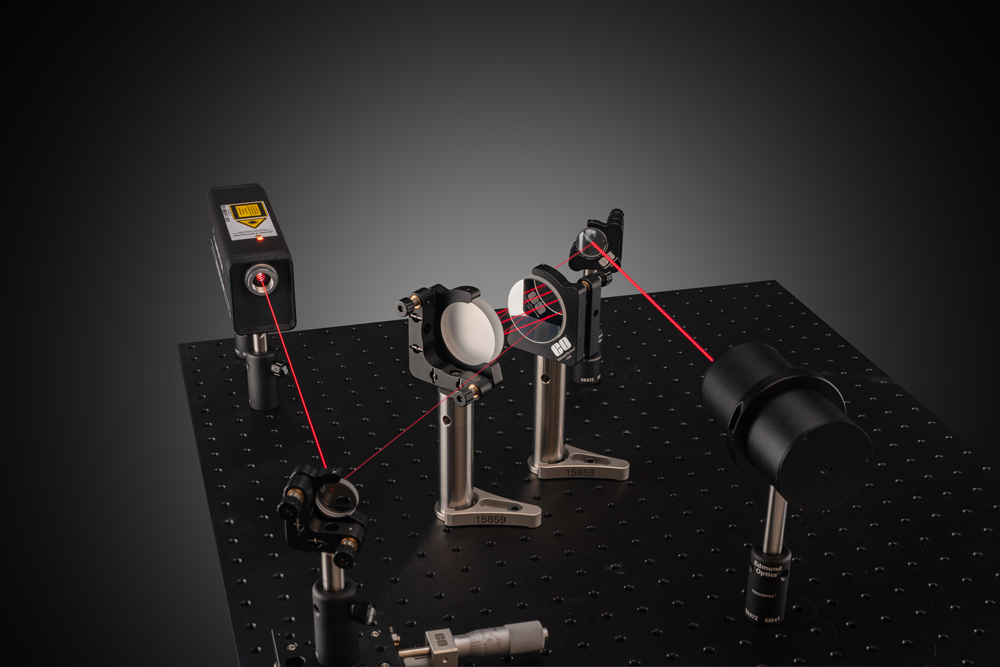
Figure 6: Highly dispersive mirrors used for ultrafast pulse compression
Challenges with White Light Interferometry
Although white light interferometry comes with plenty of benefits, it has a fair number of challenges of which users should also be aware. The use and alignment of optics are notoriously difficult, and this is a problem faced within any optical system. This only becomes more challenging within interferometry, however. The premise of constructive and destructive interference of light waves makes aligning two beams to each interfere other not only tedious, but extremely sensitive to change.
Vibrations are the number one cause of noise within an interferometric setup. These vibrations can originate from a variety of sources, anything from pressure differentials, people passing by, to other machines working in the same room. All of these factors introduce error into the system and make it more difficult to record accurate measurements.
Another common issue faced when using a WLI is working in non-visible bands of light. This makes aligning the optics that much more difficult when there is no easy way to see if the beam is going through the system properly. Using a visible guide beam can alleviate some of these difficulties, but coinciding the two beams can still prove to be difficult.
The problems listed above all impact the signal-to-noise ratio (SNR) of the interferometer. The value of SNR sets a baseline for how much noise can be allowed before the signal is no longer strong enough to record data accurately. SNR can be affected by more than the aforementioned complications, it can also be hindered by a dim source, where the signal is decreased, thus worsening the SNR. One solution is to decrease noise and increase signal by using a detector with a higher dynamic range
Off-the-Shelf Ultrafast Optics
- Ultrafast pulse compression mirrors, polarizers, lenses, windows, focusing objectives, and more
- Available for immediate shipping, ideal for quick prototyping
- Engineering support available to help select the right components for your system
Custom Ultrafast Optics Manufacturing
- Edmund Optics® designs and deposits ultrafast coatings with GDD values from -4,000 – 5,000 fs2
- Dielectric highly-reflective and anti-reflective coatings as well as ultrafast-enhanced silver coatings
- Supported by a host of in-process metrology
References
- Paschotta, R. (2008). Field guide to laser pulse generation. SPIE-International Society for Optical Engineering.
- Ellis, J. D. (2014). Field guide to displacement measuring interferometry. SPIE / International Society for Optical Engineering.
- Domas Paipulas, Rimantas Grigonis, Vaidotas Staliulionis, and Valdas Sirutkaitis "Group-delay dispersion measurements of laser mirrors using white-light interferometry", Proc. SPIE 6596, Advanced Optical Materials, Technologies, and Devices, 659614 (25 January 2007); https://doi.org/10.1117/12.726490
or view regional numbers
QUOTE TOOL
enter stock numbers to begin
Copyright 2023 | Edmund Optics, Ltd Unit 1, Opus Avenue, Nether Poppleton, York, YO26 6BL, UK
California Consumer Privacy Act (CCPA): Do Not Sell or Share My Personal Information
California Transparency in Supply Chains Act